Optical Techniques
Our group focuses on the development and application of advanced optical techniques to probe condensed matter systems. We measure quantities such as transmission and reflection coefficients, Faraday and Kerr rotation, and nonlinear optical properties. Typical experiments involve the use of light pulses with femtosecond (10-15 s) durations. Fundamental interactions in solids, which often occur on sub-nanosecond time scales, can be directly followed with these ultrafast pulses.
Pump Probe Sepctroscopy
The idea of time-resolved experiments is to use a laser pulse (“pump”) to generate a nonequilibrium population of electrons in a material. Another pulse (“probe”), delayed by a controlled amount with respect to the pump, measures the optical absorption (reflection, Kerr rotation, etc.) spectrum of the excitations induced by the first pulse. From such time-resolved spectroscopy experiments we learn important information about the recombination and scattering rates of photo-generated excitations.
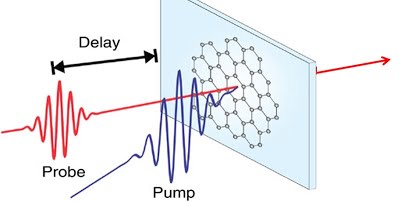
Faraday/Kerr Rotation Spectroscopy
Optical techniques using polarized light are among the most powerful methods for probing spin dynamics of electrons and holes in semiconductors. The key to optical control is the strong spin-orbit selection rules that govern absorption near the bandgap, which permit photo-generation and detection of specific spin states (and in certain special cases, specific valley states). In conventional III-V and II-VI semiconductors, the interband transition from the top valence band to the conduction band for spin up/down electrons couples only to right/left circularly polarized light. This selection rule allows to measure the spin relaxation and spin coherence by time-resolved Faraday or Kerr rotation spectroscopy.
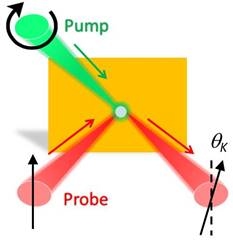
Transient Grating Spectroscopy
The conventional pump-probe technique can only measure lifetimes. Transient grating spectroscopy is a very powerful technique that can probe not only lifetimes but also transport properties. In transient grating spectroscopy, two femtosecond laser pulses are interfered non-colinearly at the semiconductor surface. If the pulses have the same polarization, interference results in a sinusoidal modulation of the laser intensity across the sample, causing an excitation of electron-hole pairs with the same spatial periodicity or wave vector. If, on the other hand, the pulses are orthogonally polarized, the laser intensity is spatially uniform, but the photon helicity varies from left to right circular polarization. For materials with optical orientation, for example GaAs, the photon helicity pattern in turn writes a sinusoidal pattern – a grating – of spin polarization. The temporal evolution of the spin polarization wave can be followed by diffracting time-delayed probe beams off the spin grating. By monitoring the decay rate of the transient spin grating as a function of wave vector, we directly measure spin lifetimes and diffusion constants.
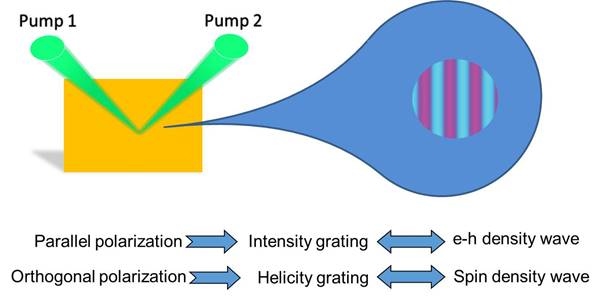
Doppler Velocimetry
While a graduate student at Berkeley, Luyi developed a novel optical technique, Doppler spin velocimetry, for probing the motion of spin polarization. This technique was applied to measure the motion of a current-driven spin texture in GaAs quantum wells. The photo-induced spin grating is injected into a moving Fermi sea, and then a time-delayed probe light is diffracted from the propagating grating of the spin density and therefore experiences a Doppler shift. By measuring the phase of the diffracting probe, the displacements of spin-valley polarization can be resolved at the level of 1 nm on a picosecond time scale.
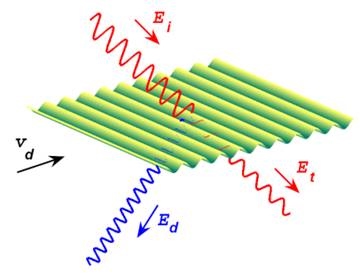
Spin Noise Spectroscopy
In addition to the pump-probe based techniques, a direct measurement of the spin relaxation can be made, even in thermal equilibrium, by using a novel and ultrasensitive technique – optical spin noise spectroscopy. This technique is based instead on “listening” (typically via optical Faraday/Kerr rotation) to the intrinsic spin fluctuations, an approach guaranteed by the fluctuation-dissipation theorem.
Two-Color Spin Noise Spectroscopy
At LANL, Luyi developed another new optical technique: two-color spin noise spectroscopy. As the name suggests, two-color spin noise spectroscopy utilizes two different probe lasers to measure spin fluctuations at different wavelengths. Correlations between these two fluctuation signals form the basis for this new methodology. This technique was applied to reveal the underlying homogeneous linewidths of individual constituents within inhomogeneously-broadened systems, e.g., electronic spins that were trapped in semiconductor quantum dots. When the two lasers have the same wavelength, they are sensitive to the same quantum dots in the ensemble and their spin fluctuation signals are correlated. In contrast, two lasers that are widely detuned from each other measure different subsets of quantum dots, leading to uncorrelated fluctuations. Measuring the noise correlation versus laser detuning directly reveals the quantum homogeneous linewidth even in the presence of a strong inhomogeneous broadening. This technique was also applied to reveal cross correlations between two types of spin species.
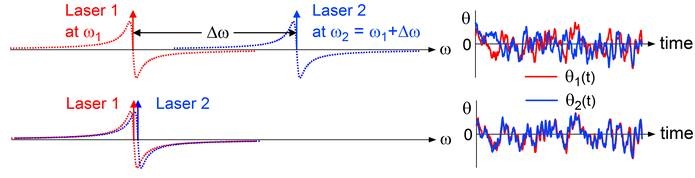
Second Harmonic Generation
We study the underlying magnetic or structural symmetry of materials with second harmonic generation. The basic idea is that we hit the sample with intensed laser pulses at the fundamental frequency and measure the second harmonic light. To select different elements of the second harmonic tensor we use polarizers before and after the sample. These define the polarization state of the fundamental and the second harmonic that reaches the detector. The information about crystal symmetry is revealed when the sample or the polarizers are rotated.
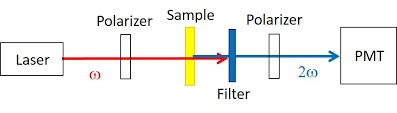
Photoluminescence Spectroscopy
Photoluminescence (PL) is light emission from any form of matter after the absorption of photons. It is a contactless, nondestructive method of probing the electronic structure of materials. Photo-excitation causes electrons within a material to move into permissible excited states. When these electrons return to their equilibrium states, the excess energy is released and may include the emission of light (a radiative process) or may not (a nonradiative process). The energy of the emitted light relates to the difference in energy levels between the two electron states involved in the transition.
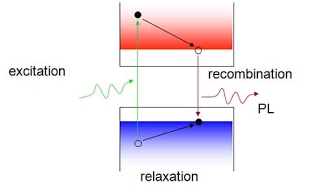